Investing in Natural Resources in a Changing World (Part IV of V)
Issues to be addressed in this research paper
For as long as I can remember, investing in natural resources has been about various industrial and precious metals, fossil fuels, timberland, etc., and not so much about lithium, graphite and other less abundant materials, but that is about to change. Quite obviously, timber will still be used to build houses and aluminium to build aeroplanes – that won’t change any time soon – but a new cohort of natural resources will dominate the headlines in the years to come, and those types of natural resources will be the focus of this research paper.
To continue reading...
In other words, if you expect this paper to provide my latest thoughts on the outlook for tin, iron ore or zinc, you will be disappointed. This paper is all about the digital revolution and which natural resources that stand to benefit from that. Climate change is the underlying factor which is driving us away from fossil fuels, and digitisation is the tool we have to address the problem.
Having said that, it is still much too early to say precisely how it will all pan out. Investing in lithium today reminds me of investing in the airline industry in the early 20th century – the first few years after the Wright brothers made their first successful flight. So much can still change, just how the aircraft of more recent times are so dramatically different from the rather primitive airplane the Wright brothers managed to get airborne in South Carolina in December 1903.
An investment in any of the natural resources referred to in this paper is therefore associated with a meaningful amount of risk. I have no particular insight into which technology (or technologies) will prevail. Nor do I have any insight into the timing of it all, although many years in this industry has taught me that scientists are almost always overly optimistic on timing, as they need to raise funds to continue their R&D programme. Timing risk is therefore one of the biggest risks when you invest in the natural resources of tomorrow, but more about that in Part V which should be with you in about a month’s time.
My story begins with lithium
Lithium is a soft, white-ish metal that almost looks like silver. It carries the symbol Li and atomic number 3. It is very light – it is actually the lightest of all alkali metals. It is either mined or it is extracted from underground pools of brine. The standard extraction technique is to evaporate water from brine, but the technique varies from country to country and from producer to producer.
Lithium is widely used in the medical profession to treat mental diseases like bipolar disorder, depression and schizophrenia. It is also used to treat alcoholism, diabetes and arthritis. In industry, for many years, the main user of lithium was the glass and ceramics industry, although it is also used elsewhere – for example in the aerospace industry where it is used in the manufacture of aircraft.
These days, the biggest user by far is the battery industry, where it is used to manufacture the rechargeable lithium-ion battery. Almost all smartphones, tablets and laptops are powered by a lithium-ion battery these days, and so are electric vehicles (EVs). See Exhibit 1 below for an overview of lithium use.
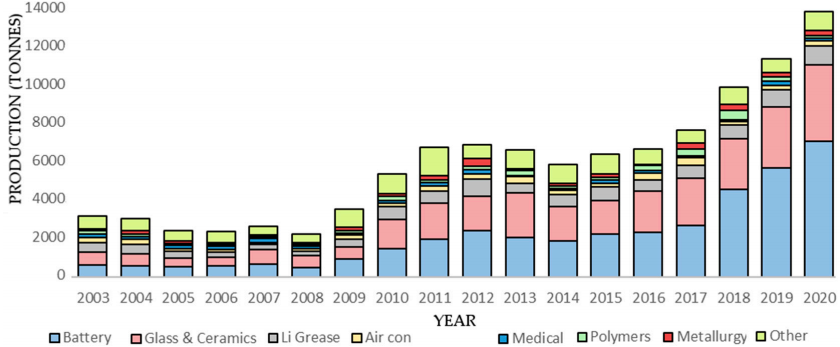
Source: mdpi.com
In the long term (10+ years), should fusion energy finally be commercialised (as I expect), demand for lithium could rise even further, as lithium is the fuel in the power plant’s reactor. I have written about this opportunity before and shall not repeat myself, but a couple of key point deserve to be reiterated.
The fusion process – converting hydrogen to helium – releases about ten million times more energy than what is released when burning the same amount of hydrogen in a conventional powerplant. While a 1,000 MW coal-fired power plant requires 2.7 million tonnes of coal per year, a fusion plant which is geared to deliver the same output will require only 250 kg of lithium annually. Technically, what happens is that, in the reactor, two hydrogen isotopes, deuterium and tritium, collide, and the fusion produces a heavier element, helium, and a neutron. You can read more about it here.
The hydrogen required comes from water (seawater will suffice), and only a few grams of fuel (lithium) are present in the plasma at any point in time. This makes the fusion reactor incredibly economical in its fuel consumption and far safer than conventional nuclear. About half a bathtub of seawater and an amount of lithium equivalent to the lithium content in one laptop battery will lead to about 200,000 kWh of electricity – about 30 years of UK per capita consumption. Therefore, once we have fusion energy, the marginal cost of generating electricity will be close to nil.
There is enough seawater to keep the show running for about six million years, and proven lithium reserves are large enough to meet expected demand for at least a few thousand years. By then, we will almost certainly have found a replacement for lithium anyway.
The declining cost of electrification
Climate change is causing more and more governments to establish programmes that favour renewable energy forms like wind and solar. One of the main problems with wind and solar (other than costs still being too high) is the fact that they are unreliable energy sources – the sun doesn’t always shine and the wind doesn’t always blow. In other words, for wind and solar to replace fossil fuels entirely, we not only need to get better at converting electricity to hydrogen, as I discussed in Part II. We also need to get much better at storing electricity, and that is why the battery technology is so important.
That said, it is not only about the technology but also about the price we can deliver that technology at. Take for example an EV battery which, on average, accounts for one-third of the cost of an electric vehicle. Eight years ago, a lithium-ion battery could be installed in an EV for $707 per kWh. Today, the comparable price is $156 – down 78% (Exhibit 2).
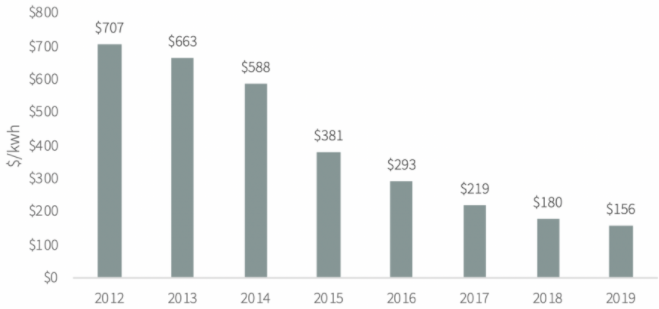
Source: Goehring & Rozencwajg, Bloomberg
According to Goehring & Rozencwajg (see for example here), industry insiders reckon EVs will reach cost parity with internal combustion engine (ICE) vehicles on a per-mile basis once lithium-ion batteries can be produced at about $100 per kwh. According to the bulls, that cost level will be reached before long, but Goehring & Rozencwajg disagree. According to their analysis, no less than 80% of the cost savings of the past eight years have come from one-time factors that cannot be repeated. For more details on their analysis, see here.
The obvious conclusion to draw is that renewable energy forms like wind and solar will not replace fossil fuels as quickly as predicted by many unless:
- battery costs drop further through innovation;
- the EV programme is subsidised; or
- the ICE motor is outlawed.
Although I agree with Goehring & Rozencwajg’s analysis, I disagree with the negative tone in their conclusion. Climate change has moved so far up the political agenda in most countries that, even if battery costs never drop to levels required to reach cost parity, a combination of subsidies and legislation will most likely continue to drive demand for EVs to new highs.
South America – the new OPEC?
For all the reasons mentioned above, it is easy to get carried away and load up on lithium in the portfolio, but there is a darker side to the story. In fact, there are two. Let’s begin with the lack of fiscal discipline in large parts of South America. Allow me to explain.
Lithium can be found on all continents. Canada, China and Australia all have significant deposits, but South America holds the biggest reserves and produces the most lithium – the majority of it in an area called the Lithium Triangle, which is where Argentina, Chile and Bolivia meet (Exhibit 3). The Lithium Triangle accounts for well over 50% of global lithium production.
My concerns have to do with the typical lack of financial acumen in South America, at least as far as public finances are concerned. If the public deficit is high and (somewhat) out of control, what will prevent a country like Argentina from flooding global markets with its lithium? Should this happen – and I would assign quite a high probability to such an outcome – lithium prices, and lithium producers all over the world, will be paying the price.
The coming battery revolution
The other issue that concerns me, and that should concern anyone with exposure to lithium, is the intense efforts in the research community to develop the battery of the future – and that is a battery which will most likely contain no lithium. According to the industry people I have consulted on this issue, demand for lithium should be robust for at least the next ten years, but they all say that, ultimately, lithium will be phased out.
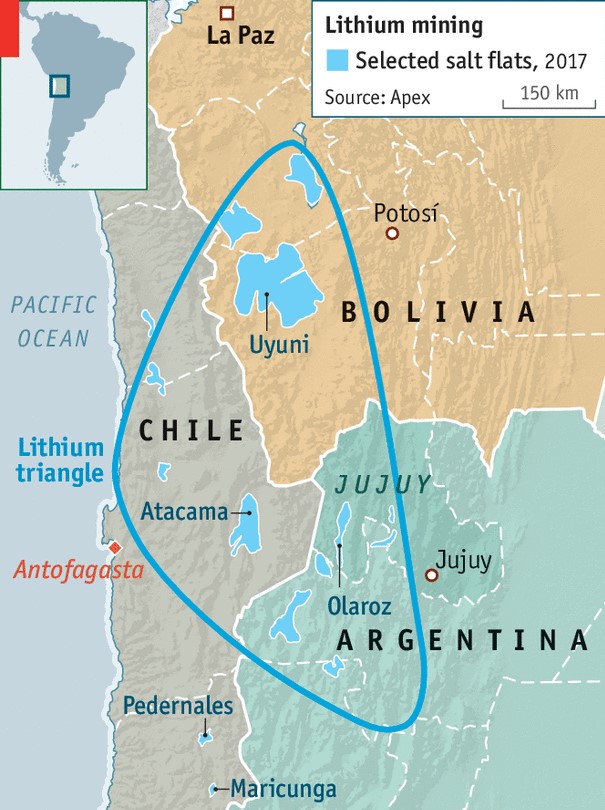
Source: The Economist
For years, battery users have been frustrated by the slow pace of battery technology improvements. In fact, one could argue that today’s lithium-ion battery is not much better than the first lithium-ion battery was, when it saw the light of day in 1991, but things are finally about to change.
Last November, Samsung issued a press release that made quite a few investors sit up and listen. Samsung said (and I paraphrase) that they have developed a so-called graphene ball, a unique material that enables a 45% increase in capacity and five times faster charging speeds compared to standard lithium-ion batteries (source: Samsung). Let’s start by exploring what graphene actually is.
The simplest way to describe graphene is that it is a single, thin layer of graphite – the soft, flaky material used in pencil lead. Graphite is an allotrope of the element carbon, meaning it possesses the same atoms but they are arranged in a different way, giving the material different properties. For example, both diamonds and graphite are forms of carbon, yet they have wildly different natures. Diamonds are incredibly strong, while graphite is brittle.
(Source: digitaltrends.com)
It has been pointed out to me that graphene is capable of transferring electricity 140 times faster than lithium, while being 100 times lighter than aluminium. Just one gram of a single layer of graphene covers the equivalent of two football pitches. This translates into an improvement in power density of a smartphone battery of 45%, which is how Samsung arrived at that number.
Samsung have since issued a press release saying that they plan to release a new smartphone powered by a graphene battery, possibly as early as this year and no later than early next year. As we all know, today’s smartphones powered by conventional lithium-ion batteries take about 90 minutes to fully charge. Samsung said that, theoretically, they should be able to reduce the charging time from 0% to 100% to just 12 minutes, but that the first generation of graphene smartphones may take as long as 25-30 minutes to fully charge.
When I first heard about Samsung’s plans, I thought the next generation graphene smartphone would contain less lithium, i.e. I thought Samsung’s new smartphone could have a negative impact on lithium prices, but that doesn’t seem to be the case. According to Hüseyin Alagöz, R&D Director of Nanografi Co. (who kindly helped me when I put this paper together), graphene is not replacing lithium but rather the graphite material in the anode of the battery. The amount of lithium compounds used in the cathode is unchanged. In other words, the use of graphene will have no impact on lithium prices.
Assuming most battery manufacturers convert to the graphene technology, once it has been fully commercialised, various estimates suggest annual demand for graphite (the raw material needed to create graphene) will quickly reach 250,000 metric tonnes (see for example this article).
There is enough graphite in deposits around the world to meet demand for many years to come (Exhibit 4), but could converting graphite to graphene become the bottleneck that slows down the conversion from conventional lithium-ion to graphene powered lithium-ion batteries?
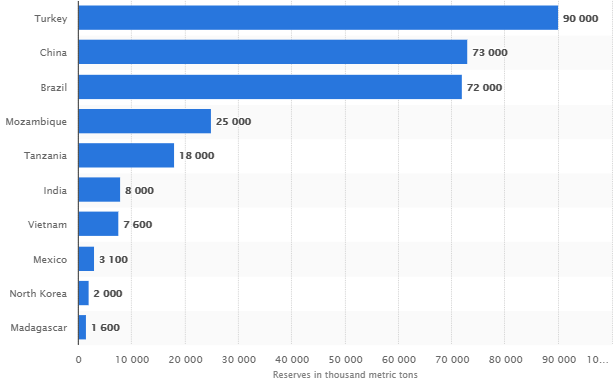
Source: Statista
Hüseyin Alagöz doesn’t think so. As he said to me:
We are one of the largest graphene manufacturers in Europe. There are several other large graphene manufacturers around the world, and new graphene companies are popping up, so we expect no graphene supply problems. The only issue is graphite supplies, as it is the key raw material going into graphene production.
What will come after graphene?
Despite the obvious advantages of using graphene in a lithium-ion powered battery, it is only an enhancement of an already existing technology – not a fundamentally new technology. Scientists are already working on the next big thing. It is therefore only fair to ask – what is likely to come after graphene? In no particular order, allow me to mention a couple of the more interesting research projects underway.
Scientists at the University of California have a new battery in the pipeline that uses sand (!) as a compound. This battery is still a lithium-ion battery but, instead of using graphite (or graphene), it uses silicon in the anodes. The sand is purified and powdered, then ground with salt and magnesium before being heated to remove oxygen, resulting in pure silicon. The test results suggest three times better performance than conventional lithium-ion batteries, and the research is so advanced that several big car manufacturers are already involved in the research.
Meanwhile, IBM has reported that they have discovered a new battery chemistry that outperforms lithium-ion batteries in a number of ways. For starters, it is cheaper to manufacture and it can charge much faster. Even better, it is entirely free from heavy metals which, if it can be commercialised, would be a first. Best of all, the materials going into this battery can all be extracted from seawater. IBM is so far down the road with this research project that it is now working with Mercedes-Benz to bring a viable EV battery to the market.
There is no shortage of fascinating battery research projects in progress but, instead of putting you to sleep, I suggest you read this paper which provides a good snapshot of current projects under developments. As you can see, and as the headline of the paper suggests, in our lifetime, we may be able to charge our batteries in seconds, re-charge them only once every few months and power them over the air.
However, as I stated earlier, these sorts of projects almost always take longer than estimated, and most of them will never come to fruition. The two research projects mentioned above seem to be advanced enough that they stand a fairly good chance of succeeding. The question is when? The feedback I got from Hüseyin Alagöz on that question was that one can fairly safely assume that lithium will be a key compound in rechargeable batteries for at least another 10-15 years.
Anything to avoid?
The obvious question to ask when a technology is changing as profoundly as the battery technology is about to is – anything to avoid from an investment point-of-view? Anything that is likely to be phased out which could – and probably would – be detrimental to the price of the material in question?
The one that sticks out is cobalt. It is used quite extensively in the cathode, i.e. in the negatively charged electrode in the battery. Cobalt is one of the least abundant and one of the most expensive compounds used when manufacturing batteries. For many years, scientists have therefore looked at alternatives to cobalt and, finally, there seems to be a breakthrough.
Researchers at University of Texas have found that that they can achieve the same results with a mix of nickel, manganese and aluminium as they can with cobalt. A mix of those three minerals will meaningfully reduce the cost of the battery, so a switch could be imminent, if the method used in Texas can be commercialised.
Secondly, if the ongoing research project at University of California that I mentioned earlier stands the test of time, graphite could potentially also be phased out down the road. I am less concerned about graphite than I am about cobalt, though, as the new graphene technology has so many other potential applications.
Nanografi Co. has provided much detail on this opportunity set – see for example here. As you can see, the application opportunities for graphene go way beyond lithium-ion batteries; which is why graphite is likely to turn into one of the key raw materials of the 21st century, graphite anodes or not.
Final few words
I haven’t mentioned copper at all yet, which I should before wrapping this paper up. The use of copper will grow immensely as transportation is electrified. Allow me to share a few data points with you. A conventional (ICE) vehicle typically contains 10-20 kg of copper depending on size and how much in the car is electrified (windows, etc.). A hybrid EV contains about 40 kg of copper, a plug-in hybrid EV about 60 kg, and an EV ‘proper’ no less than 80 kg. Even more noteworthy, an electric bus, of which there are more and more, contains a whopping 370 kg (source: Mining Technology).
There can therefore be no doubt that demand for copper will be boosted by the conversion from ICEs to EVs. The reason I am not jumping up and down in delight has to do with the cyclicality of copper. There is a reason copper is nicknamed Dr. Copper. Rising or slowing demand for copper has always been a pretty reliable leading indicator of turning points in the economy. Copper is probably the most cyclical of all the base metals and, as you know, I am not exactly bullish on the global economy as things stand. That said, could rising demand for copper from the car industry overwhelm slowing demand elsewhere? Maybe, but more work shall be required before I can reach any such conclusion.
With that observation, I shall finish this Part IV of my paper on investing in natural resources in a changing world. In Part I, I zoomed in on what is called primary energy – those fuel types that are used by power plants every day to generate electricity. In Part II – sub-titled The Fossil Fuel-Free Energy of the Future – I looked at which energy forms that are likely to ultimately replace fossil fuels, and Part III was about water and why a major crisis to do with water scarcity is looming. Finally, in this Part IV, I have tried to identify which raw materials that are most likely to benefit from electrification of everything.
That leaves only one issue to be addressed – how should investors position themselves for a largely fossil fuel-free future? That will be the question I will tackle in Part V which I aim to finish in about a month’s time.
Until then – stay safe!
Niels C. Jensen
26 August 2020